22/11/2022
In hydrogen vehicles, the hydrogen is stored in composite on-board tanks at a nominal working pressure of 35 MPa (buses) to 70 MPa (cars). When exposed to a fire, the external side of the tank wall starts to degrade and the tank gradually loses its loadbearing ability.
Due to heat transfer through the tank wall, temperature and pressure inside the tank start to grow. Eventually, when the degradation temperature front reaches a certain thickness of the tank wall where it meets the load bearing thickness of the wall, a tank burst occurs. This is accompanied by catastrophic consequences, i.e., devastating blast wave, fireball and projectiles.
The European Regulations on type-approval of hydrogen vehicles require TPRD to be installed on on-board hydrogen tanks to release their content in the event of a fire, thereby preventing the catastrophic consequences of tank rupture. When a blowdown of hydrogen through TPRD is initiated, temperature inside the tank decreases due to gas expansion. The heat transfer through the tank wall and the wall degradation are affected by two competing processes: the increase of wall temperature due to heat flux from the fire, and the decrease of the wall temperature because of the gas temperature drop inside the tank. Thus, the wall degradation front propagation slows down in conditions of blowdown, in contrast to the case of a closed vessel (Fig. 1).
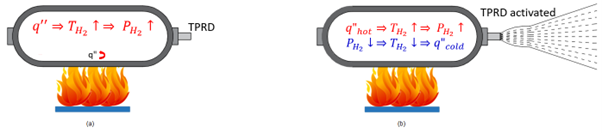
Figure 1. Tank in a fire: (a) with no blowdown (b) with release through TPRD [1].
Inherently safer design of a tank-TPRD system is a challenging task with various parameters and processes involved, including tank volume, storage pressure, TPRD release diameter, TPRD initiating time, conductive heat transfer through the wall, convective heat transfer from the fire to the wall and from the wall to the gas inside the tank, and wall material degradation due to the fire.
A physical model has been developed for the inherently safer design of a pressurised tank-TPRD system. The model accounts for the conductive heat transfer through the tank wall caused by the conjugate heat transfer at the external side of the wall (either ambient conditions or fire) and at the internal side of the tank wall between the gas and the wall. Conductive heat transfer through the tank wall is calculated by means of a one-dimensional unsteady heat transfer equation using the finite-difference method. The decomposition of the composite tank wall material (resin degradation) when the temperature exceeds the decomposition value is modelled. To calculate the heat transfer coefficient for the natural and forced convection, Nusselt number correlations are applied. The model predicts accurately the pressure and temperature dynamics inside the tank during blowdown and the blowdown time. It also makes an accurate prediction of the fire resistance rating of the tank, i.e. the time to burst of the tank in a fire and the time to leak.
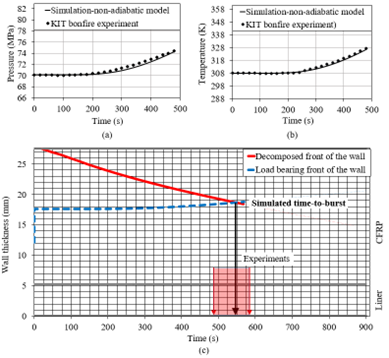
Figure 2. Comparison of model calculations with experimental data: (a) – pressure dynamics inside the tank; (b) temperature dynamics inside the tank; (c) time to burst of the tank in fire [1, 2].
Reference
[1] Dadashzadeh M, Makarov D, Kashkarov S, Molkov V. Non-adiabatic under-expanded jet theory for blowdown and fire resistance rating of hydrogen tank. In: International Conference on Hydrogen Safety (ICHS 2019), 24-26 September, Adelaide, Australia. Paper ID 182; 2019, https://hysafe.info/uploads/papers/2019/182.pdf.
[2] Molkov V, Dadashzadeh M, Kashkarov S, Makarov D. Performance of hydrogen storage tank with TPRD in an engulfing fire. International Journal of Hydrogen Energy 2021, 46: 36581-36597, https://doi.org/10.1016/j.ijhydene.2021.08.128.
For more information, please contact us by sending an email: uk@efectis.com.